Robotic Gait Therapy
Authors:
I. Vařeka 1,2; M. Bednář 1; R. Vařeková 3
Authors‘ workplace:
Rehabilitační klinika LF UK a FN Hradec Králové
1; Katedra fyzioterapie, FTK UP v Olomouci
2; Katedra přírodních věd v kinantropologii, FTK UP v Olomouci
3
Published in:
Cesk Slov Neurol N 2016; 79/112(2): 168-172
Category:
Review Article
Overview
Robotic gait therapy, one of advanced rehabilitation technologies, originally evolved as a modification of the body weight-supported treadmill therapy. At present, a range of various systems based on different principles is available, including mobile assistive exoskeletons. Neurophysiological essence of this therapy is based on the spinal cord autonomy (central pattern generators), plasticity of the central nervous system and motor learning. With respect to the evidence-based medicine, benefits of this therapy are still unclear; unambiguously positive is the reduced physical burden on a therapist. Indications must, therefore, be based on a rational consideration.
Key words:
robotics – central pattern generators – plasticity – motor learning
The authors declare they have no potential conflicts of interest concerning drugs, products, or services used in the study.
The Editorial Board declares that the manuscript met the ICMJE “uniform requirements” for biomedical papers.
Sources
1. Finch L, Barbeau H, Arsenault B. Influence of body weight support on normal human gait: development of a gait retraining strategy. Phys Ther 1991;71(11):842–55.
2. Wernig A, Müller S. Laufband locomotion with body weight support improved walking in persons with severe spinal cord injuries. Paraplegia 1992;30(4):229–38.
3. Poděbradský J, Vařeka I. Fyzikální terapie I a II. Praha: Grada 1998.
4. Nooijen CF, Ter Hoeve N, Field-Fote EC. Gait quality is improved by locomotor training in individuals with SCI regardless of training approach. J Neuroeng Rehabil 2009;6(36):36. doi: 10.1186/1743-0003-6-36.
5. Dietz V. Body weight supported gait training: from laboratory to clinical setting. Brain Res Bull 2009;78(1):I–VI. doi: 10.1016/S0361-9230(08)00410-3.
6. Mehrholz J, Pohl M. Electromechanical-assisted gait training after stroke: a systematic review comparing end-effector and exoskeleton devices. J Rehabil Med 2012;44(3):193–9. doi: 10.2340/16501977-0943.
7. Mehrholz J, Elsner B, Werner C, et al. Electromechanical-assisted training for walking after stroke. Cochrane Database Syst Rev 2013;7:CD006185. doi: 10.1002/14651858.CD006185.pub3.
8. Mehrholz J, Kugler J, Pohl M. Locomotor training for walking after spinal cord injury. Cochrane Database Syst Rev 2012;11:CD006676. doi: 10.1002/14651858.CD006676.pub3.
9. Pennycott A, Wyss D, Vallery H, et al. Towards more effective robotic gait training for stroke rehabilitation: a review. J Neuroeng Rehabil 2012;9:65. doi: 10.1186/1743-0003-9-65.
10. Pantano P, Formisano R, Ricci M, et al. Motor recovery after stroke. Morphological and functional brain alterations. Brain 1996;119(6):1849–57.
11. Blakemore S, Goodbody S, Wolpert D. Predicting the consequences of our own actions: the role of sensorimotor context estimation. J Neurosci 1998;18(18):7511–8.
12. Kwakkel G, Wagenaar R, Twisk J, et al. Intensity of leg and arm training after primary middle-cerebral-artery stroke: a randomized trial. Lancet 1999;354(9174):191–6.
13. Zeiler SR, Krakauer JW. The interaction between training and plasticity in the poststroke brain. Curr Opin Neurol 2013;26(6):609–16. doi: 10.1097/WCO.0000000000000025.
14. Richardson AG, Slotine JJ, Bizzi E, et al. Intrinsic musculosceletal properties stabilize wiping movements in the spinalized frog. J Neurosci 2005;25(12):3181–91.
15. Clarac F. Some historical reflections on the neural control of locomotion. Brain Res Rev 2008;57(1):13–21.
16. Guertin PA. The mammalian central pattern generator for locomotion. Brain Res Rev 2009;62(1):45–56. doi: 10.1016/j.brainresrev.2009.08.002.
17. Brown TG. The intrinsic factors in the act of progression in mammal. Proc R Soc B 1911;84(572):308–19.
18. Stuart DG, Hultborn H. Thomas Graham Brown (1885–1965), Anders Lundberg (1920–) and the neural control of stepping. Brain Res Rew 2008;59(1):74–95. doi: 10.1016/j.brainresrev.2008.06.001.
19. Rossignol S, Bouyer L. Adaptive mechanisms of spinal locomotion in cats. Integr Comp Biol 2004;44(1):71–9.
20. Rossignol S, Barrière G, Alluin O, et al. Re-expression of locomotor function after partial spinal cord injury. Physiology (Bethesda) 2009;24(2):127–39.
21. Wilson DM, Wyman RJ. Motor output patterns during random and rhythmic stimulation of loctus thoracic ganglia. Biophys J 1965;5(2):121–43.
22. Wilson DM. The central nervous control of flight in a locust. J Exp Biol 1961;38(2):471–90.
23. Ballion B, Morin D, Viala D. Forelimb locomotor generators and quadrupedal locomotion in the neonatal rat. Eur J Neurosci 2001;14(10):1727–38.
24. Grillner S. The motor infrastructure: from ion channels to neuronal networks. Nat Rev Neurosci 2003;4(7):573–86.
25. Kullander K. Genetics moving to neuronal networks. Trends Neurosci 2005;28(5):239–47.
26. Molinari M. Plasticity properties of CPG circuits in humans: impact on gait recovery. Brain Res Bull 2009;78(1):22–5. doi: 10.1016/j.brainresbull.2008.02.030.
27. Ivanenko YP, Poppele RE, Lacquaniti F. Distributed neural networks for controlling human locomotion: lessons from normal and SCI subjects. Brain Res Bull 2009;78(1):13–21. doi: 10.1016/j.brainresbull.2008.03.018.
28. Marchal-Crespo L, Reinkensmeyer DJ. Review of control strategies for robotic movement training after neurologic injury. J Neuroeng Rehabil 2009;16(6):20. doi: 10.1186/1743-0003-6-20.
29. Kubo K, Miyoshi T, Kanai, A, et al. Gait rehabilitation device in central nervous system disease: a review. J Robotics 2011;2011:348207.
30. Diaz I, Gil JJ, Sanchez E. Lower-limb robotic rehabilitation: literature review and challenges. J Robotics 2011;2011:759764.
31. Vařeka I, Vařeková R. Kontinuální pasivní pohyb v rehabilitaci kloubů po úrazech a operacích. Acta Chir Orthop Traumatol Cech 2015;82(3):186–91.
32. Morawietz C, Moffat F. Effects of locomotor training after incomplete spinal cord injury: a systematic review. Arch Phys Med Rehabil 2013;94(11):2297–308. doi: 10.1016/j.apmr.2013.06.023.
33. Wessels M, Lucas C, Eriks I, et al. Body weight-supported gait training for restoration of walking in people with an incomplete spinal cord injury: a systematic review. J Rehabil Med 2010;42(6):513–9. doi: 10.2340/16501977-0525.
34. Mehrholz J, Pohl M, Elsner B. Treadmill training and body weight support for walking after stroke. Cochrane Database Syst Rev 2014;1:CD002840. doi: 10.1002/14651858.CD002840.pub3.
35. Kolář P et al. Rehabilitace v klinické praxi. Praha: Galén 2010.
36. Vařeka I. Revize výkladu průběhu motorického vývoje – monokinetické stadium až batolecí období. Rehab Fyz Lek 2006;13(2):82–91.
37. Vařeka I. Revize výkladu průběhu motorického vývoje - novorozenecké období a holokinetické stadium. Rehab Fyz Lek 2006;13(2):74–81.
38. Woollacott M, Shumway-Cook A. Attention and the control of posture and gait: a review of an emerging area of research. Gait Posture 2002;16(1):1–14.
39. Woollacott M, Shumway-Cook A. Motor control: translating research into clinical practice. Philadelphia: Wolters Kluwer Health/Lippincott Williams & Wilkins 2012.
40. Collins SH, Wisse M, Ruina A. A three-dimensional passive-dynamic walking robot with two legs and knees. Int J Robotics Res 2001;20(7):607–15.
41. Collins SH, Ruina A. A bipedal walking robot with efficient and human-like gait. In: Proceedings of the 2005 IEEE International Conference on Robotics and Automation 2005, Barcelona, Spain.
Labels
Paediatric neurology Neurosurgery NeurologyArticle was published in
Czech and Slovak Neurology and Neurosurgery
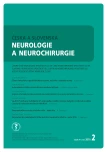
2016 Issue 2
Most read in this issue
- Ramsay-Hunt Syndrome – a Rare Manifestation of Relatively Frequent Condition
- Transient Ischemic Attack and Minor Stroke Management
- Neurosarcoidosis in a Middle-aged Man – a Case Report
- Autonomic Dysfunction and its Diagnostic Tools in Multiple Sclerosis