The Effect of Melatonin on Proliferation of Primary Porcine Cells Expressing Mutated Huntingtin
Vliv melatoninu na proliferaci primárních prasečích buněk exprimujících mutovaný huntingtin
Současné studie naznačují možnou důležitou úlohu melatoninu v Huntingtonově nemoci (HN) a jeho možné terapeutické využití při léčbě této nemoci. HN je dědičné neurodegenerativní onemocnění, které doprovází snižování hladiny melatoninu s postupem onemocnění. U normálních (nenádorových) buněk působí melatonin antiapoptoticky díky svým antioxidačním vlastnostem a schopnosti zabránit aktivaci proteinu p53. Dále melatonin zvyšuje expresi BDNF (brain derived neurotrophic factor) a dalších neuroprotektivních faktorů. Cílem této studie bylo stanovit netoxickou dávku melatoninu pro primární kožní fibroblasty izolované z transgenních miniprasat pro N‑ koncovou část lidského mutovaného huntingtinu (TgHD) a popsat efekt tohoto ošetření na tyto buňky vystavené genotoxickému stresu. Buňky byly kultivovány v médiu obohaceném různými dávkami melatoninu. Analýzou proliferačních křivek získaných mikroskopováním živých buněk v pravidelných časových intervalech jsme stanovili efekt různých koncentrací melatoninu.Ukázali jsme, že vyšší dávky melatoninu jsou pro primární prasečí buňky toxické. Je zajímavé, že TgHD buňky byly oproti kontrolním buňkám více citlivé k tímto dávkám melatoninu. Stanovili jsme efektivní dávku melatoninu a současně jsme ukázali její efekt na proliferaci u buněk vystavených genotoxickému stresu.
Klíčová slova:
Huntingtonova choroba – melatonin – mikroskopie buněk v čase – miniprasečí model –proliferační křivky – kožní fibroblasty
Autoři deklarují, že v souvislosti s předmětem studie nemají žádné komerční zájmy.
Redakční rada potvrzuje, že rukopis práce splnil ICMJE kritéria pro publikace zasílané do biomedicínských časopisů.
Authors:
P. Rausova 1,2; J. Valasek 1,2; Z. Ellederová 1; J. Motlik 1
Authors‘ workplace:
Institute of Animal Physiology and Genetics, AS CR v. v. i. and Research Centre PIGMOD, Libechov, Czech Republic
1; Department of Cell Biology, Faculty of Science, Charles University in Prague, Czech Republic
2
Published in:
Cesk Slov Neurol N 2015; 78/111(Supplementum 2): 43-48
doi:
https://doi.org/10.14735/amcsnn20152S43
Overview
According to the recent studies, melatonin might play an important role in Huntington’s disease (HD) and act as a novel therapeutic approach in the treatment of the disease. HD, the inherited neurodegenerative disorder, is accompanied by gradual melatonin reduction as it progresses. Melatonin in normal cells (non‑tumor) has the anti‑apoptotic ability due to its antioxidant property and its ability to prevent the activation of p53. Furthermore, melatonin increases the expression of BDNF (brain derived neurotrophic factor) and other neuroprotective factors. The aim of this study was to evaluate the nontoxic dose of melatonin for primary skin fibroblasts isolated from minipigs transgenic for the N‑terminal part of human mutated huntingtin (TgHD), and the effect of melatonin treatment to these cells exposed to genotoxic stress. Cells were cultured in medium supplemented with different doses of melatonin. Using time lapse microscopy, we estimated the effect of decreasing melatonin concentrations by analyzing the proliferation curves. We show that higher doses of melatonin are toxic for primary porcine fibroblasts. Interestingly, TgHD cells were more sensitive to these doses of melatonin treatment than wild type cells. We evaluated the effective dose of melatonin and demonstrated its rescue proliferative effect on porcine primary cells exposed to genotoxic stress.
Key words:
Huntington’s disease – melatonin – time lapse microscopy – minipig model – proliferation curves – skin fibroblasts
Aims of the study
Huntington’s disease (HD) represents an inherited neurodegenerative disorder lacking an effective treatment. The disease is caused by a gene‑ coding mutation for the huntingtin protein (mtHtt). HD is not only the disorder affecting the central nervous system, but it also affects the peripheral tissues, because mtHtt is widely expressed throughout the body [1]. There are many other symptoms observed in HD, in addition to neurodegeneration, like osteoporosis, testicular degeneration, and loss of muscle tissue [2].
A prominent feature of the disease is also disturbed sleep [3] caused by progressively declined melatonin levels with advancing disease [4] and significantly reduced levels of melatonin in later stages of HD [5]. The mechanism causing the melatonin secretion disruption remains unknown.
Neurodegeneration in HD is attributed to neural cell death in striatum and cortex of the brain. One of the possible therapeutic strategies targeted to neurodegeneration could represent the reduction of apoptosis [6]. Apoptosis is caused by increased levels of pro‑apoptotic protein p53. Increased levels of total p53 and also phosphorylated p53 at serine 15 are observed in brains and peripheral tissues of HD patients as well as in transgenic mouse models of HD [7,8]. Increased levels of p53 phosphorylated at serine 15 [9] are the result of DNA‑ damage accumulation in HD [10,11].
Melatonin has many physiological functions [12]. It has the ability to induce apoptosis in tumour cells while it prevents it in non‑tumour cells. It is an excellent antioxidant that acts as a free radical scavenger and increases the expression and activity of antioxidant enzymes [13]. Melatonin increases the levels of Sirt1 deacetylase in primary neurons. This positively affects the expression of BDNF (Brain Derived Neurotrophic Factor) and other neuroprotective factors and inhibits neurodegeneration in animal models of Alzheimer’s and Huntington’s disease [14]. There is evidence that melatonin mediates neuroprotection, delays the onset of the disease, and mortality in transgenic mouse models of HD [15]. These findings suggest that melatonin might act as a novel therapeutic approach in the treatment of HD. We generated a unique large animal model for HD, the minipig transgenic for the N‑terminal part of human mutated huntingtin (TgHD) [16].
The aim of the study was to follow the melatonin effect on proliferation curves of porcine primary skin fibroblasts derived from TgHD minipigs and their wild type (WT) controls. The first objective was to find the effective nontoxic concentration of melatonin treatment. Furthermore, we evaluated the effect of melatonin in TgHD and WT fibroblasts exposed to genotoxic stress.
Methods
Cell culture
Primary fibroblasts were isolated from skin grafts of miniature pigs transgenic for the N‑terminal part of human mutated huntingtin (TgHD) and their age‑ matched wild type (WT) controls with similar genetic background at the age of 36 months (pre-manifestation phase of HD). Cells were cultured in Minimal Essential Medium α (MEM α) without nucleotides (Gibco, #22561) supplemented with 10% fetal bovine serum (Sigma‑ Aldrich, #F7524) and 0.1% Gentamicin (Sigma‑ Aldrich, #G1397) under normal culture conditions (5% CO2 at 37°C). Cells were cryopreserved in the first passage in MEM α with 10% DMSO (Sigma‑ Aldrich, #D8418), 25% FBS and stored in liquid nitrogen until use. After thawing the cells in 37°C water bath, cellswere cultured as described above under normal culture conditions. All experiments were performed in the third cell passage.
Melatonin treatment
Melatonin (N‑ acetyl‑ 5- methoxytryptamine) was purchased from Sigma‑ Aldrich (#M5250) and dissolved in DMSO to obtain a stock solution at the concentration of 0,1M. Additional dilutions were done in culture medium MEM α to achieve the concentrations 10– 4M, 10– 8M, 10– 12M, 10– 14M and 10– 16M.
Neocarzinostatin treatment
Neocarzinostatin (Sigma‑ Aldrich #N9162) was added to the medium (1 : 50,000) and immediately added to the cells.
Time-lapse microscopy
Fibroblasts were seeded in the third passage on 12- well plate TPP (5 × 104 cells per well) or 24- well plate TPP (2 × 104 cells per well) and cultured six hours under normal culture conditions, while the cells were attached to the surface of the well. After incubation period, media were exchanged with corresponding media containing different doses of melatonin, melatonin and neocarzinostatin (NCS), and normal culture medium MEM α to the control cells.
Cells were imaged each hour by time‑ lapse microscopy using IncuCyte (Essen Bioscience, Welwyn Garden City, UK) with phase contrast and scan pattern 4 × 4 under normal culture conditions (5% CO2 at 37°C) for at least five days.
Data analysis
The cell confluence was calculated using the IncuCyte software (2009B, Rev2) as a mean of three replicates or two replicates (NCS treatment). To determine the maximum speed of the cell proliferation the rate of change curves, derived from cell confluence curves, was performed.
Results
In order to evaluate the optimal concentration of melatonin treatment for primary cells isolated from TgHD and age‑ matched WT miniature pigs we treated cells with different doses of melatonin ranging from 10– 4M to 10– 12M and scanned them by time‑ lapse microscopy to obtain cell growth curves (Fig. 1).
Application of melatonin at the concentration 10– 4M resulted in marked inhibition of TgHD and WT cell proliferation. Interestingly, addition of lower concentration of melatonin (10– 8M) inhibited TgHD, but did not inhibit the WT cell growth, suggesting a higher sensitivity of TgHD cells to stress. No changes of cell proliferation compared to the control were detected after incubation with the concentration of melatonin 10– 12M.
After showing that the dose 10– 12M is not toxic for primary porcine cells, we decided to measure the effect of lower doses of melatonin ranging from 10– 12M to 10– 16M (Fig. 2). Addition of melatonin at different concentrations (10– 12M, 10– 14M and 10– 16M) to the TgHD and WT cells did not inhibit cell proliferation compared to untreated control cells.
In order to choose one concentration of melatonin treatment we further examined the change in confluence derived from proliferation curves of melatonin treatment 10– 12M, 10– 14M and 10– 16M demonstrated the highest peak of the speed of cell growth at 10– 14M melatonin treatment in five from six investigated animals (Fig. 3).
Next, we investigated the effect of 10– 14M melatonin on cells exposed to genotoxic stress induced by neocarzinostatin, radiomimetic drug causing DNA double strand breaks (Fig. 4).
Neocarzinostatin caused decreasing cell growth due to DNA damage. In contrast, treating the stressed cells with melatonin 10– 14M resulted in the enhancement of the cell proliferation. This indicates that the treatment with melatonin 10– 14M of porcine cells exposed to genotoxic stress causes a rescue effect and may play a role in preventing the DNA damage.
Conclusion
In this study, we tested the effect of melatonin on porcine TgHD and WT fibroblasts exposed to genotoxic stress. The effect of melatonin on stimulation or suppression of cell division depends on its concentration and cell type examined [17], and it has not yet been published in porcine skin fibroblasts. We demonstrated that the dose of melatonin 10– 4M is toxic for primary porcine fibroblasts. Moreover, we found that TgHD cells are more sensitive to 10– 8M dose of melatonin treatment than WT cells. This shows a higher responsiveness of TgHD cells to stress. We showed that the concentrations of melatonin treatment 10– 12M 10– 14M and 10– 16M are not harmful for primary cells isolated from miniature pigs transgenic of the N‑terminal part of human mutated huntingtin. Nevertheless, based on change in confluence derived from proliferation curves, we chose the concentration of 10– 14M as an optimal concentration of melatonin treatment for porcine fibroblasts. Previous findings showed that melatonin prevents DNA-damage by promoting DNA repair [18]. Using the concentration of 10–14M, we demonstrated that melatonin treatment of porcine cells exposed to genotoxic stress caused rescue effect in WT as well as in TgHD cells, which suggests a role of melatonin in preventing DNA-damage in Huntington’s disease.
Acknowledgements
This work was funded by Program Research and Development for Innovation Ministry of Education, Youth and Sports ExAM CZ.1.05/ 2.1.00/ 03.0124, Norwegian Financial Mechanism 2009– 2014 and the Ministry of Education, Youth and Sports under Project Contract no. MSMT‑ 28477/ 2014 “HUNTINGTON” 7F14308.
The authors declare they have no potential conflicts of interest concerning drugs, products, or services used in the study.
The Editorial Board declares that the manuscript met the ICMJE “uniform requirements” for biomedical papers.
Accepted for review: 5. 10. 2015
Accepted for print: 23. 10. 2015
Mgr. Petra Rausová
Institute of Animal Physiology and Genetics
AS CR v.v.i. and Research Centre PIGMOD
Rumburska 89
277 21 Libechov
Czech Republic
e-mail: rausova@iapg.cas.cz
Sources
1. Almeida S, Sarmento‑ Ribeiro AB, Januário C, Rego AC, Oliveira CR. Evidence of apoptosis and mitochondrial abnormalities in peripheral blood cells of Huntington’s disease patients. Biochem Biophys Res Commun 2008; 374(4): 599– 603. doi: 10.1016/ j.bbrc.2008.07.009.
2. Van der Burg JM, Björkqvist M, Brundin P. Beyond the brain: widespread pathology in Huntington’s disease. Lancet Neurol 2009; 8(8): 765– 774. doi: 10.1016/ S1474‑ 4422(09)70178‑ 4.
3. Taylor N, Bramble D. Sleep disturbance and Huntington’s disease. Br J Psychiatry 1997; 171(4): 393c. doi: 10.1192/ bjp.171.4.393c.
4. Aziz NA, Pijl H, Frölich M, Schröder‑ Van Der Elst JP, Van Der Bent C, Roelfsema F et al. Delayed onset of the diurnal melatonin rise in patients with Huntington’s disease. J Neurol 2009; 256(12): 1961– 1965. doi: 10.1007/ s00415‑ 009‑ 5196‑ 1.
5. Kalliolia E, Silajdžić E, Nambron R, Hill NR, Doshi A, Frost C et al. Plasma melatonin is reduced in Huntington’s disease. Mov Disord 2014; 29(12): 1511– 1515. doi: 10.1002/ mds.26003.
6. Schapira AH V, Olanow CW, Greenamyre JT, Bezard E. Slowing of neurodegeneration in Parkinson’s disease and Huntington‘s disease: future therapeutic perspectives. Lancet 2014; 384(9942): 545– 555. doi: 10.1016/ S0140‑ 6736(14)61010‑ 2.
7. Bae B‑ I, Xu H, Igarashi S, Fujimuro M, Agrawal N, Taya Y et al. p53 mediates cellular dysfunction and behavioral abnormalities in Huntington’s disease. Neuron 2005; 47(1): 29– 41. doi: 10.1016/ j.neuron.2005.06.005.
8. Ehrnhoefer DE, Skotte NH, Ladha S, Nguyen YT, Qiu X, Deng Y et al. P53 Increases Caspase‑ 6 Expression and Activation in Muscle Tissue Expressing Mutant Huntingtin. Hum Mol Genet 2014; 23(3): 717– 729. doi: 10.1093/ hmg/ ddt458.
9. Illuzzi JL, Vickers C, Kmiec EB. Modifications of p53 and the DNA damage response in cells expressing mutant form of the protein huntingtin. J Mol Neurosci 2011; 45(2): 256– 268. doi: 10.1007/ s12031‑ 011‑ 9516‑ 4.
10. Illuzzi J, Yerkes S, Parekh‑ Olmedo H, Kmiec EB. DNA breakage and induction of DNA damage response proteins precede the appearance of visible mutant huntingtin aggregates. J Neurosci Res 2009; 87(3): 733– 747. doi: 10.1002/ jnr.21881.
11. Enokido Y, Tamura T, Ito H, Arumughan A, Komuro A, Shiwaku H et al. Mutant huntingtin impairs Ku70- mediated DNA repair. J Cell Biol 2010; 189(3): 425– 443. doi: 10.1083/ jcb.200905138.
12. Lanoix D, Lacasse AA, Reiter RJ, Vaillancourt C. Melatonin: the smart killer. The human trophoblast as a model. Mol Cell Endocrinol 2012; 348(1): 1– 11. doi: 10.1016/ j.mce.2011.08.025.
13. Richter HG, Hansell JA, Raut S, Giussani DA. Melatonin improves placental efficiency and birth weight and increases the placental expression of antioxidant enzymes in undernourished pregnancy. J Pineal Res 2009; 46(4): 357– 364. doi: 10.1111/ j.1600‑ 079X.2009.00671.x.
14. Tajes M, Gutierrez‑ Cuesta J, Ortuño‑ Sahagun D, Camins A, Pallàs M. Anti‑aging properties of melatonin in an in vitro murine senescence model: involvement of the sirtuin 1 pathway. J Pineal Res 2009; 47(3): 228– 237. doi: 10.1111/ j.1600‑ 079X.2009.00706.x.
15. Wang X, Sirianni A, Pei Z, Cormier K, Smith K, Jiang Jet al. The melatonin MT1 receptor axis modulates mutant Huntingtin‑mediated toxicity. J Neurosci 2011; 31(41): 14496– 14507. doi: 10.1523/ JNEUROSCI.3059‑ 11.2011.
16. Baxa M, Hruska‑ Plochan M, Juhas S, Vodicka P, Pavlok A, Juhasova J et al. A Transgenic Minipig Model of Huntington’s Disease. J Huntingtons Dis 2013; 2(1): 47– 68. doi: 10.3233/JHD‑ 130001.
17. Sainz RM, Mayo JC, Rodriguez C, Tan DX, Lopez-Burillo S, Reiter RJ. Melatonin and cell death: differential actions on apoptosis in normal and cancer cells. Cell Mol Life Sci 2003; 60(7): 1407–1426.
18. Santoro R, Marani M, Blandino G, Muti P, Strano S. Melatonin triggers p53Ser phosphorylation and prevents DNA damage accumulation. Oncogene 2012; 31(24): 2931–2942.
Labels
Paediatric neurology Neurosurgery NeurologyArticle was published in
Czech and Slovak Neurology and Neurosurgery
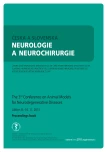
2015 Issue Supplementum 2
Most read in this issue
- The Libechov Minipig as a Large Animal Model for Preclinical Research in Huntington’s disease – Thoughts and Perspectives
- Buccal Epithelial Cells as Potential Non‑ invasive Materials for the Monitoring of Mitochondrial Disturbances to Track Huntington‘s Disease Progression – a Pilot Study
- Telemetry Physical Activity Monitoring in Minipig’s Model of Huntington’s Disease
- Grunting in a Genetically Modified Minipig Animal Model for Huntington’s Disease – Pilot Experiments