Differences in the Modulation of Cortical Activity in Patients Suffering from Upper Arm Spasticity Following Stroke and Treated with Botulinum Toxin A
Rozdíly v modulaci kortikální aktivity u pacientů po cévní mozkové příhodě s reziduální spasticitou ruky léčených botulotoxinem A
Cíl:
Cílem naší fMR studie bylo lokalizovat změny aktivace mozkového kortexu u pacientů po cévní mozkové příhodě s reziduální spasticitou ruky léčených botulotoxinem A (BoNT).
Soubor a metodika:
Bylo vyšetřeno 34 pacientů po prodělané cévní mozkové příhodě s reziduální spasticitou ruky; z tohoto počtu vyšetřených byly nezávisle analyzovány dvě homogenní podskupiny. Skupina A byla tvořena 4 mladými pacienty (2 muži, 2 ženy, věk 25 ± 3,4 let, rozptyl 22–31 let) s plegií ruky. Skupina B byla tvořena 5 staršími pacienty (4 muži, 2 ženy, věk 67 ± 11,1 let, rozptyl 54–80) s parézou ruky. Mozkový motorický systém byl mapován pomocí funkční magnetické rezonance (fMR) během provádění motorické úlohy postiženou končetinou (skupina A: myšlený pohybu prstů; skupina B: skutečný pohyb prstů). Vyšetření bylo opakováno dvakrát, vždy před a čtyři týdny po aplikaci BoNT. Skupina B byla vyšetřena ještě potřetí s odstupem 11 týdnů od aplikace BoNT. Změna spasticity byla hodnocena pomocí modifikované Ashworthovy škály (MAS).
Výsledky:
Léčba BoNT snížila spasticitu u všech pacientů; hodnoceno čtyři týdny po aplikaci. fMR před aplikací BoNT ukazovalo abnormně rozsáhlou a bilaterální aktivaci korových oblastí během motorické úlohy. Po aplikaci BoNT došlo k dočasné a částečné normalizaci obrazu aktivace. Kontrast pre- > post-BoNT prokázal signifikantní snížení aktivace v zadním cingulu/precuneu (skupina A) a dorzolaterálním prefrontálním kortexu (skupina B).
Závěr:
Naše výsledky naznačují, že i struktury mimo klasický senzorimotorický systém se uplatňují u postiktální spasticity.
Klíčová slova:
cévní mozková příhoda – spasticita ruky – funkční magnetická rezonance – botulotoxin
Authors:
T. Veverka 1; P. Hlustik 1,2; P. Hok 1; Z. Tomasova 1; P. Otruba 1; M. Král 1; Z. Tudos 2; A. Krobot 3; R. Herzig 1; P. Kaňovský 1
Authors‘ workplace:
Faculty of Medicine and Dentistry, Palacký University and University Hospital, Olomouc
Department of Neurology
1; Faculty of Medicine and Dentistry, Palacký University and University Hospital, Olomouc
Department of Radiology
2; Faculty of Medicine and Dentistry, Palacký University and University Hospital, Olomouc
Department of Physiotherapy
3
Published in:
Cesk Slov Neurol N 2013; 76/109(2): 175-182
Category:
Original Paper
Autoři deklarují, že v souvislosti s předmětem studie nemají žádné komerční zájmy.
Redakční rada potvrzuje, že rukopis práce splnil ICMJE kritéria pro publikace zasílané do biomedicínských časopisů.
Overview
Aim:
The aim of our functional magnetic resonance (fMRI) study was to localize the changes of cerebral cortex activation in stroke patients suffering from upper limb spasticity and treated with botulinum toxin A (BoNT).
Materials and methodology:
34 patients suffering from upper limb post-stroke spasticity were examined; 9 of these patients were grouped into two homogenous subgroups that allowed independent further analysis. Group A consisted of four patients (2 males, 2 females; aged 25.5 ± 3.4 years, range 22–31 years) who suffered from hand plegia. Group B consisted of five patients (4 males, 1 female; aged 67.0 ± 11.1 years, range 54–80) who were able to perform real finger movement. The change of arm spasticity was assessed by using the modified Ashworth scale (MAS). fMRI was performed during imaginary movement (group A) or real movement of the impaired hand (group B). fMRI sessions were performed before (W0) and four weeks (W4) after BoNT treatment. Group B underwent additional fMRI 11 weeks (W11) after BoNT application.
Results:
BoNT treatment decreased arm spasticity in all patients assessed 4 weeks following the BoNT injection. fMRI pre-BoNT treatment showed extensive and bilateral task-related activation of cortical areas. Following the BoNT treatment, temporary and partial shift towards normal distribution of activity occurred. The pre>post-BoNT contrast revealed a significant decrease in activation of the posterior cingulate/precuneus region (group A) and dorsolateral prefrontal cortex (group B).
Conclusion:
Our results imply that structures outside the traditional sensorimotor system may play a role in the relief of post-stroke spasticity.
Key words:
stroke – spasticity – functional magnetic resonance imaging – botulinum toxin
Introduction
Impairment of corticofugal tracts (tr. corticospinalis) in ischemic stroke leads to motor deficit presented by the majority of the surviving patients [1]. Up to two thirds of stroke survivors experience impaired function and spasticity of the upper limb [2]. A lesion in the sensory or motor circuits typically causes greater weak-ness of the wrist and fingers than proximal shoulder muscles [3]. Most of the motor function recovery or improvement occurs within 3–6 months post stroke and recovery is expected to plateau six months post stroke [4]. Three recovery mechanisms are suggested [5]. Restitution includes processes that follow successful recanalisation of the occluded vessel and function restitution in the penumbra zone. Substitution includes processes likely related to brain plasticity [6–12]. Compensation comprises functional improvement achieved by modification of the movement pattern.
Brain plasticity means that the structure and function of the brain are continually reorganizing [13]. Reorganization of the sensory-motor maps is caused by afferent inputs, i.e. interactions with the environment, its changes, learning etc. Brain plasticity after stroke is associated with substitution by undamaged areas in primary sensory and motor cortex and other cortical areas. Performance of an active motor task after stroke is associated with lateral and posterior displacement of activity in M1, probably into S1 [9,14]. The displacement occurs progressively over time in association with recovery of hand function [15].
The degree of muscle weakness is crucial in determining the degree of movement deficit [16,17]. Other motor (especially spasticity) and non-motor (e.g. neglect, sensory impairment, emotional and speech-related difficulties) may also contribute. Spasticity frequently causes problems with common daily activities, hygiene, physical therapy, and nursing care [18]. A prospective study found spasticity prevalence to be 38% one year after stroke [19]. Focal spasticity is currently successfully treated with botulinum toxin [20,21].
There is growing evidence that BoNT treatment can relieve focal spasticity not only due to the peripheral site of action [22]. We hypothesized that besides the well-known neuromuscular junction site of action, BoNT treatment can relieve focal spasticity through dynamic changes at multiple levels of the motor system, presumably including the cerebral cortex. These processes should be reflected in changes of cortical activation during motor or mental tasks, as assessed using functional magnetic resonance imaging (fMRI).
The aim of our fMRI study was to localize the changes in cerebral cortex activation in stroke patients suffering from upper limb spasticity and treated with BoNT.
Patients and methods
The study was approved by the institutional ethics committee and all subjects gave written consent for the study in accordance with the Helsinki Declaration.
A total of 34 patients who were in the chronic phase of ischemic stroke and manifesting with distal upper arm spasticity, were enrolled (tab. 1).
Four patients discontinued the study protocol and were excluded from further analysis. Twenty five patients underwent the complete protocol with 3 fMRI sessions; 5 patients underwent an incomplete protocol with 2 fMRI sessions. All enrolled patients suffered from unilateral distal arm weakness with spasticity following ischemic stroke at point 1+ or higher of the modified Ashworth scale (MAS). Exclusion criteria were the duration of post--stroke period less than 3 months, severe cognitive deficit and severe depression assessed using the MMSE and Zung scales [23,24], as these could alter participation in the study; and magnetic resonance imaging exclusion criteria.
Nevertheless, the whole group of enrolled patients was very heterogeneous. Patients differed in several characteristics, especially age, severity of neurological impairment and degree of spasticity. To obtain more homogeneous subgroups suitable for group analysis, we considered age and severity of neurological impairment as two main variables affecting both motor system plasticity and functional MRI task selection. Two subgroups that emerged using this classification were analyzed independently.
The first subgroup (group A) consisted of 4 young patients (2 males, 2 females; aged 25.5 ± 3.4 years, range 22–31 years) who suffered from hand plegia (movement disability) and performed imagined finger movement as the functional MRI activation task [25]. The patients’ characteristics are listed in tab. 2.
The second subgroup (group B) consisted of 5 elderly patients (4 males, 1 female; aged 67.0 ± 11.1 years, range 54–80) who were able to perform real finger movement; this type of task allowed visual control and correction of finger movement. These patients also underwent an additional fMRI session 3 months following the BoNT application, when the effect on muscle fibers diminished [26]. The patients’ characteristics are listed in tab. 3.
Patients were studied using a previously published protocol [25]. Spasticity was evaluated during clinical examination using the Modified Ashworth Scale [27]. The assessments were done at Week 0, when patients were screened, enrolled and injected with BoNT, then at Week 4, four weeks following the injection of BoNT (when BoNT effect is assumed to be maximal) and at Week 11 (only group B), 3 months after the BoNT injection, when the effect on muscle fibers ceased.
Similarly to behavioral assessments, functional MRI examinations were done at Week 0, Week 4 and Week 11 (only group B).
Task
The task itself was a sequential finger movement or mental movement simulation in Roland’s paradigm using the impaired hand. Patients with hand plegia (group A) first practiced the task with their healthy fingers and, when in the MRI scanner, they were asked to imagine performing the same movement with their impaired fingers. Patients with preserved movement of the impaired hand moved their fingers (in the sequential Roland’s paradigm) under visual control of a technician. In the MRI scanner, the task was performed with eyes closed, and the beginning and end of the active block were signaled verbally (start/stop) in MR-compatible headphones. The block paradigm timing was 15 s on (active task) – 15 s off (rest). Each experimental run consisted of 12 repetitions of the same task-rest block pairs, for a total of 6 minutes. Each participant had two experimental runs with the impaired hand.
Treatment
The patients were treated with BoNT A injections into muscles of the affected arm at week 0. Treatment was injected using EMG guidance (Medtronic Keypoint, Alpine Biomed ApS, Denmark) and electrical muscle stimulation. The following muscles were always treated: the flexor carpi ulnaris muscle (FCU), the flexor carpi radialis muscle (FCR), the flexor digitorum superficialis muscle (FDS), and the flexor digitorum profundus muscle (FDP). When there was a need for additional injections into other muscles of the upper limb, they were injected using the same technique; the following muscles were injected: m. brachioradialis (BR) in one patient, m. biceps brachii (BB) in one patient, m. pronator teres (PT) in two patients. The dose of BoNT (Botox®) was 50 U (100 U in biceps brachii) per muscle. The effect of the BoNT treatment was tested using both clinical (MAS) and imaging assessments.
Data acquisition
Magnetic resonance imaging data were acquired on 1.5 Tesla scanners (Avanto and Symphony, Siemens, Erlangen, Germany) with a standard head coil. The MR imaging protocol covered the whole brain with 30 axial slices 5 mm thick, including anatomical T1-weighted images to provide an immediate overlay with functional data, fluid-attenuated inversion recovery (FLAIR) images to visualize brain lesions, functional T2*-weighted (BOLD) images during task performance and rest, and a high-resolution 3D anatomical scan (MPRAGE). BOLD images were acquired with gradient echo EPI, TR/TE = 2,500/40 ms, FOV 220 mm, to provide 3.4 × 3.4 × 5 mm resolution. 144 images were acquired per each 6-minute functional run. A subject’s head was immobilized with cushions to ensure maximum comfort and to minimize head movement.
Analysis
fMRI data processing was carried out using FEAT (FMRI Expert Analysis Tool) Version 5.98, part of FSL (FMRIB‘s Software Library, www.fmrib.ox.ac.uk/fsl). The following pre-statistics processing was applied: motion correction using MCFLIRT [28]; slice-timing correction using Fourier-space time-series phase-shifting; non-brain removal using BET [29]; spatial smoothing using a Gaussian kernel of full width at half-maximum (FWHM) 10 mm; grand-mean intensity normalization of the entire 4D dataset by a single multiplicative factor; high-pass temporal filtering (Gaussian-weighted least-squares straight line fitting, with sigma = 15.0 s). Time-series statistical analysis was carried out using FILM with local autocorrelation correction [30]. Registration to high resolution structural and/or standard space images was carried out using FLIRT [28,31].
Higher-level analysis was carried out using FLAME (FMRIB‘s Local Analysis of Mixed Effects) stage 1 and 2 with automatic outlier detection [32–34]. Z (Gaussianized T/F) statistic images were thresholded using a corrected cluster significance threshold of p = 0.05 [35]. In group B, the longitudinal 3-session design permits separation of the physiotherapy effects, expected to be gradually developing over time, from the transient effect of BoNT. The treatment effect at session 2 (W4) may be decomposed into a transient BoNT effect and a progressive effect of time and physiotherapy using specific post-hoc linear contrasts, comparing the weighted average of sessions 1 and 3 and session 2, using temporal distance from session 2 as the weights: (7 × S1 + 4 × S3)/11 versus S2.
Results
Behavioral
BoNT treatment decreased arm spasticity across all patients measured 4 weeks following the BoNT injection.
In group A, the mean MAS scores were 3.5 (SD= .57) at Week 0, 1.38 (SD= .49) at Week 4 and the mean MAS change from baseline was 2.1 (P= .0013, one-sided paired t-test).
In group B, the mean MAS scores were 2.3 (SD= .67) at Week 0, 1.7 (SD= .27) at Week 4 and the mean MAS change from baseline was 0.6 (P= .0724, one-sided paired t-test). In group B, the mean MAS score at Week 11 was 2.2 (SD= .45) and did not significantly differ from Week 0 (MAS change 0.1, p = 0.352, one-sided paired t-test).
Imaging – group mean
Functional MRI during impaired hand movement or during imagined movement before BoNT treatment showed an extensive bilateral network of active areas, including the contralesional motor cortex, supplementary motor area, bilateral premotor cortices, bilateral superior parietal lobe, precuneus/posterior cingulate, bilateral basal ganglia and ipsilesional cerebellum (fig. 1a).
Imaging – treatment effects
After the BoNT-treatment, both groups manifested reduction of previously widespread activation towards normal spatial distribution of activity into the midline and contralesional sensorimotor cortex (fig. 1b).
fMRI 11 weeks after the BoNT application (group B) showed a similar pattern of activation as the session prior to the BoNT treatment (fig. 1c).
In the group of patients with arm plegia (group A) the pre>post-BoNT contrast revealed a significant decrease (p < 0.05) in activation of the posterior cingulate/precuneus region close to the midline, centered on the Montreal Neurological Institute (MNI) coordinates –8, –48, 0 (fig. 2).
In the group of patients with preserved finger movement (group B), the pre>post-BoNT contrast revealed a significant decrease (p < 0.05) in activation of the following contralesional cortical areas: dorsolateral prefrontal cortex, inferior frontal gyrus, and postcentral gyrus (fig. 3). Local maxima for between-session contrast reflecting BoNT treatment effect in group B are shown in tab. 4.
Discussion
Cerebral plasticity plays an important role in post-stroke recovery. Functional imaging studies showed that motor recovery is associated with massive recruitment of areas in the motor system early after the stroke. Initial increase in bilateral sensorimotor activity patterns normalize during recovery [36–39]. Alleviation of the extent and bilateral movement-related activity is recovery-dependent: patients demonstrating poor motor recovery continue to activate a number of primary and non-primary motor regions more than those demonstrating good recovery [40,41].
Processes of cerebral plasticity may not be purely beneficial but can in fact even impair residual function (maladaptive plasticity) as reported in conditions such as dystonia, phantom limb pain and allodynia [42–45]. Development of spasticity after stroke may also be related to such maladaptive processes.
At present, BoNT is a well-established part of multimodal antispastic treatment. It has been proven that BoNT acts peripherally. However, there also is some evidence that BoNT acts through a supraspinal mechanism and can even affect cortical reorganization [21,46,47].
Most of the previous fMRI studies described changes in task-related cortical activity after specific rehabilitative treatment, e.g. constraint-induced therapy [41]. Only a few studies reported cortical changes after BoNT injections into the spastic muscles [25,26,48].
In our research, we focused on the relationship between dynamic changes in movement-related brain activation and alleviation of spasticity induced by BoNT treatment.
In both our experiments, functional MRI examination done prior to the treatment showed widespread and bilateral activation during the motor task. The most significant regions of activation were found in the contralesional motor cortex, supplementary motor area, bilateral premotor cortices, bilateral superior parietal lobe, precuneus/posterior cingulate, bilateral basal ganglia and ipsilesional cerebellum. Imagery of finger movements (group A) evoked activation in the same cortical areas as those associated with performed movements (group B), similar to that shown previously [49].
We can assume that this pretreatment activation might be a uniform pattern of the lesioned brain as a form of maladaptation due to increased pathological proprioceptive afferentation (via Ia fibers) that is associated with spasticity.
As expected, BoNT-derived alleviation of spasticity was found in both groups and it was more prominent in the group of completely plegic patients with higher MAS before treatment. Also, the BoNT treatment-related reduction of abnormal extent and bilateral activation was observed in both groups. We extended the protocol of the first experiment by adding a third fMRI session (group B) at the time when BoNT effect on muscle fibers waned. BoNT-off data revealed a wide recruitment of cortical areas similar to that before the treatment. This approach allowed us to distinguish the BoNT-related effect from other mechanisms that could affect the results, e.g., spontaneous functional recovery or rehabilitation-related changes. In addition, all patients were in the chronic post-ischemic stroke stage, when activation patterns are stable [41].
In the periphery, BoNT affects intrafusal fibers as well as extrafusal ones and thus alters pathological sensory inputs to the CNS by blocking the neuromuscular junction of the gamma motor neurons. This blockade leads to a reduction of Ia afferent signals and indirectly inhibits the pre-existing feedback-driven execution mode [50]. This might be the mechanism through which the BoNT treatment reduces maladaptive plasticity. The hypothesis of central reorganization following BoNT treatment is supported by studies using neurophysiologic and imaging methods in patients with focal dystonia [51–53].
In both our experiments, decreased activation was found not only in the traditional motor regions but surprisingly also in regions that are not usual components of the motor system. The strongest hotspots were identified within the posterior cingulate cortex in group A and the dorsolateral prefrontal cortex (DLPFC) in group B.
The posterior cingulate (group A), consisting of Brodmann areas 29, 30, 23, and 31, is a component of the limbic system and has been associated with functions such as working memory, encoding of visuomotor tasks and extrapersonal space and global attention [54–56].
The posterior cingulate cortex has been frequently mentioned in connection with the default network [57]. The dorsolateral prefrontal cortex (group B) is thought to be an association area that includes parts of the superior and middle frontal gyrus, a minor portion of the inferior frontal gyrus in Brodmann areas 9 and 46 [58]. DLPFC has been associated with such functions as working memory, decision making and executive functions [59–61]. Both posterior cingulate and DLPFC also contribute to motor performance and motor skill [62,63]. DLPFC has dense connections with cingulate cortices as was confirmed in previous studies [64,65].
On one hand, the localization of changes in both groups falls in the same functional category of “extended” motor system, i.e., areas that are not active during performance of common motor tasks but are related to either complex aspects of motor planning or adaption to extended motor skill learning or injury of motor structures. On the other hand, there are obvious differences between the groups. It is rational to associate them primarily with the basic differences between the groups, i.e., age and severity of paresis. We presume that motor control in the elderly patients with preserved voluntary hand movement resembles healthy subjects. DLPFC is a frontal association area, hierarchically superior to the premotor cortex and is activated in healthy subjects while planning and performing complex motor tasks, even though it has no direct connections to spinal motor neurons and acts through hierarchically lower premotor and motor areas. From this perspective, DLPFC is closer to the traditional cortical motor system (areas with corticospinal projections). On the other hand, the posterior cingulate/precuneus area that manifested changes in the group of younger patients with hand plegia, is also mentioned in association with the motor system but at a qualitatively different level. This greater functional “distance” could be linked to inability to perform real voluntary hand movements in the presence of subcortical lesion of the corticospinal tract and, consequently, abnormal movement control. In such a situation, the motor network may engage anatomically and functionally more “distant” cortical areas. Unfortunately, these speculations are difficult to support with ancillary data as, for example, analogous studies in stroke patients with hand plegia treated for spasticity are presently unavailable. However, since both our pilot groups involved rather a small number of patients, the results should be interpreted with caution and generalization of our results to the whole clinical population is impossible. Nevertheless, we assume that the above mentioned areas may play a role in performing movement in situation when the traditional motor structures are engaged in the processing of abnormal proprioceptive input from the spastic muscles of a paretic extremity.
The authors declare they have no potential conflicts of interest concerning drugs, products, or services used in the study.
The Editorial Board declares that the manuscript met the ICMJE “uniform requirements” for biomedical papers.
Tomas Veverka, MD
Department of Neurology
Faculty of Medicine and Dentistry
Palacký University and University Hospital
I. P. Pavlova 6
775 20 Olomouc
e-mail: veverka.tomas@seznam.cz
Accepted for review: 28. 3. 2011
Accepted for print: 8. 2. 2013
This work was supported by the IGA Ministry of Health Czech Republic grant number NT13575-4/2012 and by institutional support of UH Olomouc.
Sources
1. Hendricks HT, van Limbeek J, Geurts AC, Zwarts MJ. Motor recovery after stroke: a systematic review of the literature. Arch Phys Med Rehabil 2002; 83(11): 1629–1637.
2. Jørgensen HS, Nakayama H, Raaschou HO, Vive-Larsen J, Støier M, Olsen TS. Outcome and time course of recovery in stroke. Part I: Outcome. The Copenhagen Stroke Study. Arch Phys Med Rehabil 1995; 76(5): 399–405.
3. Colebatch JG, Gandevia SC. The distribution of muscular weakness in upper motor neuron lesions affecting the arm. Brain 1989; 112(3): 749–763.
4. Page SJ, Gater DR, Bach-Y-Rita P. Reconsidering the motor recovery plateau in stroke rehabilitation. Arch Phys Med Rehabil 2004; 85(8): 1377–1381.
5. Dobkin BH, Carmichael TS. Principles of recovery after stroke. In: Barnes M, Dobkin B, Bogousslavsky J (eds). Recovery after Stroke. Cambridge: Cambridge University Press 2005.
6. Weiller C, Chollet F, Friston KJ, Wise RJ, Frackowiak RS. Functional reorganization of the brain in recovery from striatocapsular infarction in man. Ann Neurol 1992; 31(5): 463–472.
7. Ward NS, Brown MM, Thompson AJ, Frackowiak RS. Longitudinal changes in cerebral response to proprioceptive input in individual patients after stroke: an fMRI study. Neurorehabil Neural Repair 2006; 20(3): 398–405.
8. Rijntjes M, Weiller C. Recovery of motor and language abilities after stroke: the contribution of functional imaging. Prog Neurobiol 2002; 66(2): 109–122.
9. Calautti C, Baron JC. Functional neuroimaging studies of motor recovery after stroke in adults: a review. Stroke 2003; 34(6): 1553–1566.
10. Bütefisch CM, Davis BC, Wise SP, Sawaki L, Kopylev L, Classen J et al. Mechanisms of use-dependent plasticity in the human motor cortex. Proc Natl Acad Sci U S A 2000; 97(7): 3661–3665.
11. Rossini PM, Calautti C, Pauri F, Baron JC. Post-stroke plastic reorganisation in the adult brain. Lancet Neurol 2003; 2(8): 493–502.
12. Cramer SC. Functional imaging in stroke recovery. Stroke 2004; 35 (11 Suppl 1): 2695–2698.
13. Pascual-Leone A, Amedi A, Fregni F, Merabet LB. The plastic human brain cortex. Annu Rev Neurosci 2005; 28: 377–401.
14. Weiller C, Ramsay SC, Wise RJ, Friston KJ, Frackowiak RS. Individual patterns of functional reorganization in the human cerebral cortex after capsular infarction. Ann Neurol 1993; 33(2): 181–189.
15. Jaillard A, Martin CD, Garambois K, Lebas JF, Hommel M. Vicarious function within the human primary motor cortex? A longitudinal fMRI stroke study. Brain 2005; 128(5): 1122–1138.
16. Kamper DG, Fischer HC, Cruz EG, Rymer WZ. Weakness is the primary contributor to finger impairment in chronic stroke. Arch Phys Med Rehabil 2006; 87(9): 1262–1269.
17. Ada L, O’Dwyer N, O’Neill E. Relation between spasticity, weakness and contracture of the elbow flexors and upper limb activity after stroke: an observational study. Disabil Rehabil 2006; 28(13–14): 891–897.
18. Barnes MP. Medical management of spasticity in stroke. Age Ageing 2001; 30 (Suppl 1): 13–16.
19. Watkins CL, Leathley MJ, Gregson JM, Moore AP, Smith TL, Sharma AK. Prevalence of spasticity post stroke. Clin Rehabil 2002; 16(5): 515–522.
20. Ward AB, Aguilar M, De Beyl Z, Gedin S, Kanovsky P, Molteni F et al. Use of botulinum toxin type A in management of adult spasticity – a European consensus statement. J Rehabil Med 2003; 35(2): 98–99.
21. Rosales RL, Dressler D. On muscle spindles, dystonia and botulinum toxin. Eur J Neurol 2010; 17 (Suppl 1): 71–80.
22. Antonucci F, Rossi C, Gianfranceschi L, Rossetto O, Caleo M et al. Long-distance retrograde effects of botulinum neurotoxin A. J Neurosci 2008; 28(14): 3689–3696.
23. Folstein MF, Folstein SE, McHugh PR. „Mini-mental state“. A practical method for grading the cognitive state of patients for the clinician. J Psychiatr Res 1975; 12(3): 189–198.
24. Zung WW. A self-rating depression scale. Arch Gen Psychiatry 1965; 12: 63–70.
25. Senkarova Z, Hlustik P, Otruba P, Herzig R, Kanovsky P. Modulation of cortical activity in patients suffering from upper arm spasticity following stroke and treated with botulinum toxin A: an fMRI study. J Neuroimaging 2010; 20(1): 9–15.
26. Tomasova Z, Hlustik P, Kral M, Otruba P, Herzig R, Krobot A et al. Cortical activation changes in patients suffering from post-stroke arm spasticity and treated with botulinum toxin A. J Neuroimaging 2011. DOI: 10.1111/j.
27. Bohannon RW, Smith MB. Interrater reliability of a modified Ashworth scale of muscle spasticity. Phys Ther 1987; 67(2): 206–207.
28. Jenkinson M, Bannister P, Brady M, Smith S. Improved optimization for the robust and accurate linear registration and motion correction of brain images. Neuroimage 2002; 17(2): 825–841.
29. Smith SM. Fast robust automated brain extraction. Hum Brain Mapp 2002; 17(3): 143–155.
30. Woolrich MW, Ripley BD, Brady M, Smith SM. Temporal autocorrelation in univariate linear modeling of fMRI data. Neuroimage 2001; 14(6): 1370–1386.
31. Jenkinson M, Smith S. A global optimisation method for robust affine registration of brain images. Med Image Anal 2001; 5(2): 143–156.
32. Beckmann CF, Jenkinson M, Smith SM. General multilevel linear modeling for group analysis in fMRI. Neuroimage 2003; 20(2): 1052–1063.
33. Woolrich MW, Behrens TE, Beckmann CF, Jenkinson M, Smith SM. Multilevel linear modelling for fMRI group analysis using Bayesian inference. Neuroimage 2004; 21(4): 1732–1747.
34. Woolrich M. Robust group analysis using outlier inference. Neuroimage 2008; 41(2): 286–301.
35. Worsley KJ. Statistical analysis of activation images. In: Jezzard P, Matthews PM, Smith SM (eds). Functional MRI: an introduction to methods. Oxford: Oxford University Press 2001: 251–270.
36. Small SL, Hlustik P, Noll DC, Genovese C, Solodkin A. Cerebellar hemispheric activation ipsilateral to the paretic hand correlates with functional recovery after stroke. Brain 2002; 125(7): 1544–1557.
37. Calautti C, Leroy F, Guincestre JY, Marié RM, Baron JC. Sequential activation brain mapping after subcortical stroke: changes in hemispheric balance and recovery. Neuroreport 2001; 12(18): 3883–3886.
38. Feydy A, Carlier R, Roby-Brami A, Bussel B, Cazalis F, Pierot L et al. Longitudinal study of motor recovery after stroke: recruitment and focusing of brain activation. Stroke 2002; 33(6): 1610–1617.
39. Marshall RS, Perera GM, Lazar RM, Krakauer JW, Constantine RC, DeLaPaz RL. Evolution of cortical activation during recovery from corticospinal tract infarction. Stroke 2000; 31(3): 656–661.
40. Ward NS, Brown MM, Thompson AJ, Frackowiak RS. Neural correlates of outcome after stroke: a cross-sectional fMRI study. Brain 2003; 126(6): 1430–1448.
41. Johansen-Berg H, Dawes H, Guy C, Smith SM, Wade DT, Matthews PM. Correlation between motor improvements and altered fMRI activity after rehabilitative therapy. Brain 2002; 125(12): 2731–2742.
42. Pujol J, Roset-Llobet J, Rosinés-Cubells D, Deus J, Narberhaus B, Valls-Solé J et al. Brain cortical activation during guitar-induced hand dystonia studied by functional MRI. Neuroimage 2000; 12(3): 257–267.
43. Flor H. Remapping somatosensory cortex after injury. Adv Neurol 2003; 93: 195–204.
44. Maihöfner C, Handwerker HO, Birklein F. Functional imaging of allodynia in complex regional pain syndrome. Neurology 2006; 66(5): 711–717.
45. Kanovsky P. Dystonia: a disorder of motor programming or motor execution? Mov Disord 2002; 17(6): 1143–1147.
46. Kaňovský P, Streitová H, Daniel P, Hekerlová R, Bareš M, Dufek J. Dlouhodobá remise cervikální dystonie navozená léčbou botulotoxinem A – signál možného ovlivnění centrálního dystonického mechanismu? Cesk Slov Neurol N 1998; 61/94(2): 123–134.
47. Kanovsky P, Streitova H, Dufek J, Znojil V, Daniel P, Rektor I. Change in lateralization of the P22/N30 cortical component of median nerve somatosensory evoked potentials in patients with cervical dystonia after successful treatment with botulinum toxin A. Mov Disord 1998; 13(1): 108–117.
48. Manganotti P, Acler M, Formaggio E, Avesani M, Milanese F, Baraldo A et al. Changes in cerebral activity after decreased upper-limb hypertonus: an EMG-fMRI study. Magn Reson Imaging 2010; 28(5): 646–652.
49. Roth M, Decety J, Raybaudi M, Massarelli R, Delon-Martin C, Segebarth C et al. Possible involvement of primary motor cortex in mentally simulated movement: a functional magnetic resonance imaging study. Neuroreport 1996; 7(7): 1280–1284.
50. Rosales RL, Arimura K, Takenaga S, Osame M. Extrafusal and intrafusal muscle effects in experimental botulinum toxin-A injection. Muscle Nerve 1996; 19(4): 488–496.
51. Kanovsky P, Bares M, Streitova H, Klajblova H, Daniel P, Rektor I. Abnormalities of cortical excitability and cortical inhibition in cervical dystonia Evidence from somatosensory evoked potentials and paired transcranial magnetic stimulation recordings. J Neurol 2003; 250(1): 42–50.
52. Gelb DJ, Yoshimura DM, Olney RK, Lowenstein DH, Aminoff MJ. Change in pattern of muscle activity following botulinum toxin injections for torticollis. Ann Neurol 1991; 29(4): 370–376.
53. Ceballos-Baumann AO, Sheean G, Passingham RE, Marsden CD, Brooks DJ. Botulinum toxin does not reverse the cortical dysfunction associated with writer’s cramp. A PET study. Brain 1997; 120(4): 571–582.
54. Mesulam MM, Nobre AC, Kim YH, Parrish TB, Gitelman DR. Heterogeneity of cingulate contributions to spatial attention. Neuroimage 2001; 13(6): 1065–1072.
55. Bollinger J, Rubens MT, Zanto TP, Gazzaley A. Expectation-driven changes in cortical functional connectivity influence working memory and long-term memory performance. J Neurosci 2010; 30(43): 14399–14410.
56. Bledowski C, Rahm B, Rowe JB. What „works“ in working memory? Separate systems for selection and updating of critical information. J Neurosci 2009; 29(43): 13735–13741.
57. Uddin LQ, Kelly AM, Biswal BB, Xavier Castellanos F, Milham MP. Functional connectivity of default mode network components: correlation, anticorrelation, and causality. Hum Brain Mapp 2009; 30(2): 625–637.
58. Rajkowska G, Goldman-Rakic PS. Cytoarchitectonic definition of prefrontal areas in the normal human cortex: II. Variability in locations of areas 9 and 46 and relationship to the Talairach Coordinate System. Cereb Cortex 1995; 5(4): 323–337.
59. Halsband U, Lange RK. Motor learning in man: a review of functional and clinical studies. J Physiol Paris 2006; 99(4–6): 414–424.
60. Galea JM, Albert NB, Ditye T, Miall RC. Disruption of the dorsolateral prefrontal cortex facilitates the consolidation of procedural skills. J Cogn Neurosci 2010; 22(6): 1158–1164.
61. Vanderhasselt MA, De Raedt R, Leyman L, Baeken C. Role of the left DLPFC in endogenous task preparation: experimental repetitive transcranial magnetic stimulation study. Neuropsychobiology 2010; 61(3): 162–168.
62. Jäncke L, Shah NJ, Peters M. Cortical activations in primary and secondary motor areas for complex bimanual movements in professional pianists. Brain Res Cogn Brain Res 2000; 10(1–2): 177–183.
63. Milton J, Solodkin A, Hlustík P, Small SL. The mind of expert motor performance is cool and focused. Neuroimage 2007; 35(2): 804–813.
64. Petrides M, Pandya DN. Dorsolateral prefrontal cortex: comparative cytoarchitectonic analysis in the human and the macaque brain and corticocortical connection patterns. Eur J Neurosci 1999; 11(3): 1011–1036.
65. Paus T, Castro-Alamancos MA, Petrides M. Cortico-cortical connectivity of the human mid-dorsolateral frontal cortex and its modulation by repetitive transcranial magnetic stimulation. Eur J Neurosci 2001; 14(8): 1405–1411.
Labels
Paediatric neurology Neurosurgery NeurologyArticle was published in
Czech and Slovak Neurology and Neurosurgery
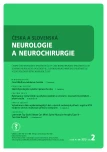
2013 Issue 2
Most read in this issue
- Creutzfeldt-Jacob disease
- Spinocerebellar Ataxia 7 – a Case Report
- Lyme Borreliosis as a Cause of Bilateral Neuroretinitis with Pronounced Unilateral Stellate Maculopathy in a 8-Year Old Girl
- Electrophysiological Examination of the Pelvic Floor